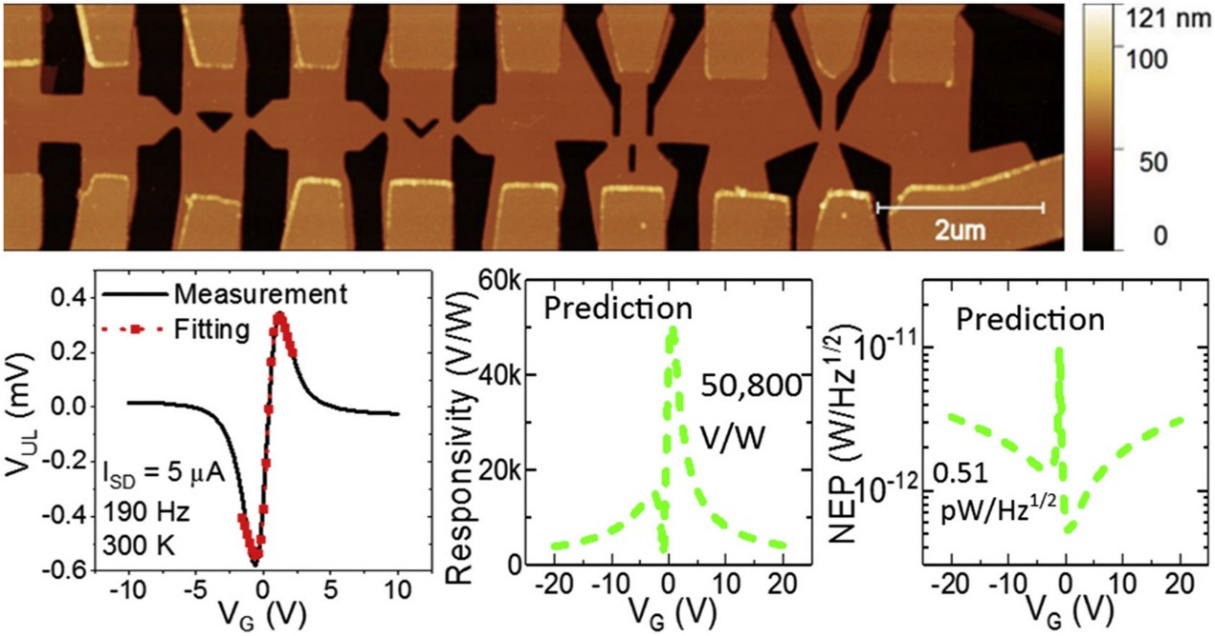
The research group of Prof. Aimin Song of the School of Microelectronics has made new progress in the theory of graphene ballistic rectifiers. The result has been published in Carbon (IF: 8.82) with title “Graphene ballistic rectifiers: Theory and geometry dependence”. Prof. Song is the corresponding author and the co-first author is Prof. Jiawei Zhang from the School of Microelectronics, Shandong University and Dr. Joseph Brownless from the University of Manchester.
Since graphene was first experimentally recorded in 2004, it has been subject to a huge degree of research for potential applications in electronics and in many other fields. In particular, its high room-temperature mobility exceeding 200,000 cm2/Vs makes graphene attractive for use in high-frequency electronics. In order to overcome the obstacle of a lack of a bandgap, researchers have performed many studies on planar and vertical tunneling devices, graphene nanoribbons, and different surface modifications. There are very few devices however which utilize the intrinsic long mean-free path, which is a result of its high carrier mobilities. The ballistic rectifier (BR) is a planar device which rectifies current due to ballistic transport of carriers and its asymmetric structure, and it differs from conventional diodes in that it requires no doping junctions or barriers. The graphene ballistic rectifier (GBR) is therefore capable of harnessing the high mobility and long mean-free path of graphene and has been demonstrated to operate beyond 650 GHz. The GBR does not require a bandgap to operate, and its dimensions are in the same order of magnitude as or less than the mean-free path, so most carrier transport is ballistic and carriers are generally only scattered at the graphene edges. The GBR resembles a bridge rectifier in operation but operates with zero threshold because it does not need to overcome any built-in potential. This property is very important for high-frequency detectors because the input signals are typically very low in amplitude and it means that the device need not be biased, eliminating a source of electrical noise, which is a particularly critical performance-limiting factor. Electrical noise is further reduced by the GBR structure, in which the input direction of current flow is orthogonal to the output measurement and hence the signals are mostly decoupled.
Prof. Song’s group previously reported two distinct structures of BRs, one of which uses a triangular scattering center and the other instead uses angled source and drain contacts, although by no means do these represent the only possible configurations of four-terminal graphene ballistic rectifiers. However, the only theory of operation yet developed is for BRs with triangular scattering centers in a 2D electron gas formed in a semiconductor heterostructure, where only one type of carrier is important for transport. In graphene however, the zero bandgap means the coexistence of electrons and holes unless the graphene is heavily biased away from the carrier neutrality point. Due to the operational mechanism of the GBR, the direction of the output depends on the charge of the majority carrier, therefore in the vicinity of the carrier neutrality point, the presence of both electrons and holes decreases the output magnitude. So far, the theory of operation for four-terminal graphene ballistic rectifiers remains to be developed.
In this work, Prof. Song present the operating mechanism and analytical theory of the GBR, developed using the Büttiker-Landauer formalism and considering coexistence of electrons and holes. Based on this theory, four GBRs with different structures are demonstrated, and their output voltages agree well with the derived equation. The research group then use the derived theory to predict the characteristics of GBRs with different geometries, noting the unexpected improvement of responsivity when there is a large difference in carrier mobilities. This work offers a guideline to the future design of GBRs and may broaden the potential applications of GBRs in THz detection. The developed theory may also be applied to other graphene-based nanodevices where both types of carriers may coexist.
In the past few years, Prof. Song and his research group has made a series of advances in the graphene-based planar rectifiers, such as the first graphene-based full-wave self-switching rectifiers (Nanotechnology, 30, 36, 2019, IF:3.55), the graphene THz detectors (Nano Letters, 17, 11, 7015, 2017, IF:11.24), graphene ballistic nano-rectifier with very high responsivity (Nature Communications, 7, 11670, 2016, IF:12.12), and ballistic rectifiers using CVD graphene (Carbon, 84, 124, 2015, IF:8.82). The work was supported by the North-West Nanoscience Doctoral Training Center, EPSRC Grants EP/N021258/1, EP/G03737X/1, and EP/L01548X/1, the Royal Society grants IEC∖R2∖170155 and NA170415, National Key Research and Development Program of China Grants 2016YFA0301200 and 2016YFA0201800, and National Natural Science Foundation of China Grants 11374185 and 11304180.